Research Article - Volume 3 - Issue 4
Biomarkers of some commercial lotion and shampoo based-pesticides toxicity in male mice
Khaled A Osman1*; Nagat M Aly2; Nabila S Ahmed1
1Department of Pesticide Chemistry & Technology, Faculty of Agriculture, El-Shatby, P.O. Box 21545, Alexandria University, Alexandria, Egypt.
2Central Agricultural Pesticide Lab., Agriculture Research Center, Sabahia, Alexandria, Egypt.
Received Date : May 20, 2023
Accepted Date : July 04, 2023
Published Date: July 11, 2023
Copyright:© Khaled A. Osman 2023
*Corresponding Author : Khaled A. Osman, Department of Pesticide Chemistry and Toxicology, Faculty of Agriculture, Pesticide Chemistry and Technology department, Alexandria University, Aflaton St., EL-Shatby-21545, Alexandria, Egypt.
Email: khaled-osman@alexu.edu.eg
DOI: Doi.org/10.55920/2771-019X/1486
Abstract
The effects of Quick (0.5% malathion) and Licid (0.6% tetramethrin with 2.4% piperonyl butoxide) lotions, and Newcid shampoo (1% permethrin) applied on the whole-body of male mice was investigated. Newcid and Licid treatments induced signs of toxicity characterized by tremor, salivation and diarrhea, while Quick lotion produced signs of intoxication characterized by nausea, vomiting, seizures, convulsions, salivation and lacrimation. Quick treatment significantly inhibited brain Acetylcholinesterase (AChE), liver Aspartate Aminotransferase (AST) and Alanine Aminotransferase (ALT), and serum ALT, while brain Gamma Aminobutyric Acid (GABA) level and liver ATPase activity significantly increased. Licid-treated mice showed marked inhibition in brain AChE, serum ALT, and liver AST and ALT, whereas significant increases in the activities of serum AST and liver AT Pase were recorded. Also, Newcid significantly decreased ALT activities in serum and liver, and the levels of GABA and glutamic acid in brain, while liver ATPase activity significantly increased compared to control values. The tested pediculicides decreased liver Acid Phosphatase (ACP) activity by 5-12%, while liver Carboxylesterase (CarE) activity decreased by 6 and 13% when mice treated with Newcid and Licid, respectively. In conclusion, the alteration of some biomarkers from the control values denotes biochemical impairment and reflect the side effects of lotions and shampoos based-pesticides to mice. Since there are similarities between different vertebrate groups, disorders observed in mice may indicate risks to children. More studies are necessary to evaluate the risks of these pediculicides to children.
Keywords: Lice; Pediculicides; Mice; Biomarkers; Side effects; Risk.
Introduction
Head lice (Pediculus humanus capitis) are considered as the most prevalent parasitic infestation of humans in the developed and developing countries, where the parasitic insects live on the scalp and neck hairs of humans and feed on human blood, causing social distress, discomfort, anxiety, and embarrassment [1,2]. The most likely targets for invasion by head lice are kindergarten and school-aged children between 3 and 11 years and occur commonly each year in all socioeconomic statuses [3-5]. In the United States, each year about 6-12 million kids lose their lost school days due to the infestation of head lice and treatment costs of more than US 500 million/year [6]. There are many products on the markets formulated as creams, shampoos or lotions based- insecticides and acaricides with different pharmacological mode of actions to control ectoparasites on humans such as lice and mites. The proper application of these products must be emphasized to ensure that the treatment is effective with avoiding reinfestation with head lice and spreading it by contact to close people [7]. Pyrethroids and lindane which are used for non-ovicidal therapies, require 2-3 treatments to control lice, while malathion which is used for ovicidal therapy, requires 1-2 treatments [8]. Pediculicides used to control head lice on humans should be regulated and approved by the national health authority for this specific use, and it should be applied according to the manufacturer’s instructions [9], to achieve successful treatment sufficient quantity to wet all the hair [10].
Permethrin and tetramethrin belong to type I synthetic pyrethroids which lack a-cyano moiety [11], that are extensively used in public health, and veterinary applications [12] as well as play a vital role in personal care products such as shampoo and mosquito-repellent perfume [13]. Permethrin 1% is still the first line treatment of head lice [14]. Although pyrethroids are classified as safe insecticides, several of which were found to induce neurotoxicity, where they affect the mammalian central nervous system [15]. Moreover, pyrethroids interact with sodium channels and inhibit its reactivation, increase depolarization of the neuron and affect the release of neurotransmitters [16-20]. Concluded that pyrethroids produce adverse impacts on neurodevelopment which are likely to occur at exposure levels in the general population, and the implementation to protect vulnerable population groups such as pregnant women and children is warranted.
Malathion belongs to Organophosphorus Insecticides (OPs) that is extensively used through the whole world, especially in developing countries to control or eradicate disease-inducing arthropods targeted by public health programs, animal ectoparasites, head lice in humans, and household insects [21-23]. The use of OPs has increased considerably due to their low toxicity with low persistence in the mammalian system compared to organochlorine pesticides [24,25]. OPs, in addition to their intended effects like control of insects or other pests, are often found even to affect non-target organisms including humans [26]. It is well known that OPs exert their toxicity through inhibition of Acetylcholinesterase (AChE), which leads to the Accumulation of Acetylcholine (ACh) and the subsequent activation of cholinergic muscarinic and nicotinic receptors [27,28]. Also, OPs are known to exert several toxicological effects such as delayed neurotoxicity and/or polyneuropathy as a result of inhibition of neuropathy target esterases [29]. The instructions for using pediculicides should be periodically tested to document to prove the levels of lice resistant to the active ingredients of these pediculicides and to the formulated products [9]. Alternative topical pediculicides including formulations containing malathion and benzyl alcohol are available now, but resistance still makes anxiety [30].
Many parents often repeatedly and prophylactically apply pediculicides to their children without realizing the hazardous effect of their misuse or overuse. Many factors such as the activity of ovicide, its toxicity, and coasts affect the selecting of a pediculicide. Close attention must be given to the safety of children, and parents and caregivers often require education regarding proper application of such products to avoid toxicity and ensure treatment success [7]. Unfortunately, shampoo and lotion based-pesticides have been associated with childhood acute leukemia [31], neurotoxicity [32] and mutation [33]. In view of the increasing hazard posed by commercially available lotions and shampoos based-pesticides to children used to control lice and nits in children. The present study aimed to investigate the effect of lotion and shampoo formulations which have been successfully commercialized in public health in Egypt to control lice and nits on children namely, Newcid shampoo (1% permethrin), and Licid (0.6% tetramethrin and 2.4% piperonyl butoxide) and Quick (0.5% malathion) lotions on the activities of Acetylcholinesterase (AChE), Aspartate Aminotransferase (AST) and Alanine Aminotransferase (ALT), Adenosine Triphosphatase (ATPase), Acid Phosphatase (ACP) and Carboxylesterase (CarE) as well as the levels of Gamma Aminobutyric Acid (GABA) and glutamic acid in different organs of male mice following whole body hair treatment as biomarkers of toxicity.
Materials and Methods
Chemicals
The tested lotion and shampoo formulations were purchased from a pharmacy located in Alexandria city, Egypt. Newcid shampoo containing 1% permethrin (3-phenoxybenzyl (1RS)-cis-trans-3- (2,2-dichlorovinyl) -2,2-dimethyl cyclopropanecarboxylate), Licid lotion containing 0.6% tetramethrin (cyclo-1-ene-1,2- dicaroximidomethyl-2,2-dimethyl-3- (2-methylprop-1-enyl) cyclopropanecarboxylate) and 2.40% of the synergist piperonyl butoxide (5- [2- (2- butoxy-ethoxy) ethoxymethyl] -6-propyl -1,3-benzodioxole), and Quick lotion containing 0.5% malathion (diethyl (dimethoxy thiophosphorylthio) succinate) manufactured by Chemical Industries Development, Miser Company for Pharmaceutical Industries, and Arab Drug Company, Egypt, respectively. Acetylthiocholine iodide and 5,5`-dithiobis (2-nitrobenzoic acid) were purchased from Sigma Chemical Company, while sodium p-nitrophenyl phosphate, p-nitrophenyl butyrate, Gamma Aminobutyric Acid (GABA), glutamic acid, and Adenosine Triphosphat (ATP) were obtained from BDH Chemical Company. Kits used for the determination of Aspartate Aminotransferase (AST) and Alanine Aminotransferase (ALT) activities were purchased from Scalvo Diagnostics Kit (Italy). Other chemicals used in the present study were of the highest grade commercially available.
Animal care, treatment, and enzyme assays
The housing of the male Swiss Albino mice (Mus musculus), handling, breeding and bioassay were conducted with the guidelines of the Institutional Animal Care and Use Committee (IACUC), Alexandria University, Egypt. Mice weighing 25.0±3.0 g (two months old) were obtained from the High Institute of Public Health, University of Alexandria, Egypt. The animals were randomly divided into 4 groups (5 mice each) and housed in stainless steel cages, kept under laboratory conditions (25±2 ºC, 50-70% humidity and 12h light: 12h dark cycle) for one week prior to the beginning of the experiments with free access to tap water and standard mice chow ad labium. The first three groups were treated with Newcid, Licid and Quick. The application of the formulations was done according to the manufacturer's instructions, where the lotion or shampoo was applied to saturate the hair on the whole body, left for 1 hr, and then washed off with tap water. The application was repeated after three days; then mice were sacrificed 24 hrs after the last treatment. Mice in the fourth group were treated with tap water and kept as control. r. Symptoms of toxicity in the treated groups were recorded. Blood was obtained from a cardiac puncture of each animal using a syringe, transferred into non-heparinized tubes, and then centrifuged for at 3,000 xg for 10 min and 4 °C in a HS-21 (MSE) centrifuge. Serum was withdrawn and for the determination of biochemical parameters. Animals in each group were sacrificed by decapitation, then liver and brain were quickly removed, separately weighed and homogenized in ice cold saline solution (10% w/v) using polytron Homogenizer (Tekmar tissumizer). The homogenates were centrifuged at 10,000xg for 20 min at 4 °C using HS-21 (MSE) cooling centrifuge and the supernatant (microsomal plus soluble factions) was used for assaying the biochemical parameters. Brain Acetylcholinesterase (AChE) was assayed by the method of [34] using acetylthiocholine iodide as a substrate and the activity was expressed as acetylthiocholine hydrolyzed/min/mg protein, while g-GABA and glutamic acid levels in brain were measured using the chromatographic method [35] and the levels were expressed as mg/mg wet tissue. Liver Adenosine Triphosphatase (ATPase) was determined using adenosine triphosphate as a substrate and the specific activity was expressed as mmole of inorganic phosphate produced/min/mg [36]. The activities of aminotransferases (Aspartate Aminotransferase, AST and Alanine Aminotransferase, ALT) in serum and liver were assayed as described by the commercial assay kits using the method of [37] and their activities were expressed as unit/mg protein. The activities of Carboxylesterase (CarE) and acid phosphatase (ACP) in livers were determined using p-nitrophenyl butyrate and p-nitrophenyl phosphate as the substrates according to the methods of [38,39], and activities were expressed as nmole and mmole of p-nitrophenol produced/min/mg protein, respectively. Protein contents in serum and supernatants were measured using bovine serum albumin as the standard [40].
Statistical analysis
Data were calculated as mean±Standard Deviation (SD) analyzed using Analysis Of Variance (ANOVA) and Least Significant Difference (LSD) multiple comparison tests were used to compare between treatments at level of 0.05 (p< 0.05). Tukey posthoc test was adopted to ascertain the significant differences. The statistical package of Costat Program was used for all chemometric calculations [41].
Results and discussion
There are many antilice formulations that are successfully commercialized for the control of lice and nits in the local market of Egypt. Treatment of pediculosis relies widely on the use of topical insecticides, with a neurotoxic mode of action, such as topical pyrethroids or malathion [42]. Currently 3 pesticides namely, permethrin, tetramethrin and malathion are available by prescription and used in numerous formulations. Permethrin and tetramethrin belong to synthetic pyrethroids and formulated as shampoo and lotion, respectively, while malathion is an OP and formulated as lotion. The neurotoxicity and biochemical effects of Newcid shampoo (1% permethrin), Licid lotion (0.6% tetramethrin with 2.4% piperonyl butoxide) and Quick lotion (0.5% malathion) on male mice were investigated.
Signs of toxicity
During the study period, there was no mortality in any treated group. Newcid and Licid treatments induced signs of toxicity characterized by tremors, salivation and diarrhea and Licid was more potent for inducing these signs. The co-administration of pyrethroids with piperonyl butoxide increased the insecticidal efficacy as well as toxicity, where the LD50 value decreased [43]. The present results are in parallel with many investigators who reported that the exposure to non a-cyano pyrethroids such as permethrin and tetramethrin induced clinical signs of toxicity in mammals such as whole-body tremor (T syndrome), hyperactivity, loss control of bodily movements, paraesthesia, spasms, and paralysis [44-46]. In addition, permethrin (1% cream rinse) induced signs of intoxication characterized by burning, tingling, numbness, and erythema [47].
In the present study, mice treated with Quick lotion (0.5% malathion) produced signs of intoxication due to inhibition of AChE and accumulation of ACh resulted in cholinergic symptoms characterized by nausea, vomiting, seizures, convulsions, salivation and lacrimation [27,28]. No lethality was observed during this study and treated animals were completely recovered from the intoxication after 3-4 hrs in case of Newcid and Licid treatments and 6 hrs after Quick treatment because body can rapidly metabolize permethrin, tetramethrin, and malathion to water-soluble metabolites and excreted in urine [48]. Permethrin and tetramethrin are relatively more quicker than malathion to be metabolized in the body by enzymes that are not substantially present in insects with minimal percutaneous absorption and favourable safety profiles, so they are considered to be less toxic to humans [47-50). On the contrary, malathion used in lice treatments, which is potentially more potent, may present more chance for toxicological effects on humans [51].
Biochemical effects of pediculicides
Effect on brain AChE
AChE is an enzyme that breaks down the neurotransmitter ACh at the synaptic cleft so that the subsequent nerve impulse can be transmitted across the synaptic gap [52], and the inhibition of this enzyme can be used as a biological marker of OPs and carbamate poisonings [53]. Data presented in Table 1 point out that whole brain AChE activities were significantly inhibited by 33 and 21% of control value after Quick and Licid lotions treatments, respectively, while the brain AChE activity in mice treated with Newcid non-significantly changed from control value. Quick contains malathion, which acts as anti-AChE [24] and has been linked to the dysfunction of several organ systems [23,28]. The inhibition of AChE in mice previously treated with Quick indicates that malathion found in this formula penetrated the skin of treated mice and reached the brain and consequently neurotransmission was disturbed leading to a number of neurological disorders such as paralysis and eventual death [54]. Also, Licid was found to inhibit AChE although it is not the main target of pyrethroids because the formula of Licid contains PB (2.40%) besides tetramethrin, and the methylenedioxyphenyl ring in the structure of PB is documented to act as anti-esterases [55] and may cause carcinogenic and teratogenic effects in mammals [56,57]. On the other hand, some pyrethroids such as deltamethrin were found to induce a remarkable increase in the activity of AChE in various brain regions of rats [58,59], while others such as allethrin and cyhalothrin had no effect on cortex, hippocampus and striatum AChE activities in rat [60].
The high degree of efficiency of formulae containing malathion and used against head lice has been attributed to the presence of malathion 0.5%, as well as a high concentration of isopropyl alcohol (78%) and terpineol (12%) [61], where this alcohol can penetrate the insect cuticle results in denaturation of proteins in eggs and adults and dehydrate eggs [4], while terpineol has ovicidal and pediculicidal activity [62] and acts by affecting AChE and octopamine receptors, causing neuronal hyperactivity and death in insects [63,64]. However, when 0.5% of malathion was applied to the scalp of volunteers, it is absorbed and rapidly metabolized by tissue A-esterases and Carboxylesterases (CarEs) to inactive metabolites (monocarboxylic and dicarboxylic acid derivatives of malathion) that are subsequently excreted in the urine [65,66,48], and the administered doses were not sufficient to induce the inhibition of AChE activity [67]. Only with overwhelming doses of malathion, liver metabolizes malathion to the active metabolite, malaoxon [65], which is good inhibitor for AChE [24].
Effect on GABA and glutamate neurotransmitters
Glutamic acid and GABA are neurotransmitters that mediate the excitatory and inhibitory transmission in the CNS of vertebrates and invertebrates, respectively [68-70]. Also, glutamate plays an important role in the induction of hippocampal long-term potentiation, a phenomenon considered to underlie the neurochemical basis of learning and memory [71]. Data in Table 1 show that Quick lotion treatment significantly increased the level of GABA in the whole brain by 69% compared to untreated mice, with non-significant changes in glutamic acid levels. The disruption of the balance between glutamic acid and GABA by Quick containing malathion, noted in the present study, may preferentially affect sodium channels on GABAergic, thereby increasing the release of GABA and in turn decreasing the release of glutamate. On the other hand, Newcid treatment inhibited the release of GABA and glutamic acid levels of mice brain by 23 and 17%, respectively, while Licid treatment produced nonsignificant reduction either in the levels of GABA or glutamic acid of mice brain, where the percentages of reduction were 8 and 10, respectively. It was documented that pyrethroids act on GABA-gated chloride channels [72]. The present results are in parallel with the results of [73] who reported that deltamethrin induced decreases and increases in GABA and glutamate release, respectively. The reduction in GABA levels obtained in the present study after Newcid application may be due to the inhibition of glutamic acid decarboxylase following the local formation of 2 keto-4-pentenoic acid [74]. The enzymes involved in the conversion of 2 keto-4-pentenoic acids are widely distributed within the brain [75]. In general, the imbalance between GABA and glutamate is linked with some neurodevelopmental disorders [75-78].
Effect on transaminases
Transaminases (AST and ALT) are the most imperative, sensitive and specific marker of liver function [54] and have an important role in the biological processes that catalyze the transferring of amino groups from amino acids to oxo-acids. They are responsible for detoxification processes and biosynthesis of energetic macromolecules for different essential functions [79]. AST and ALT are common markers of liver injury, where the determination of activities in serum are used as a routine tool in the diagnosis of diseases [80]. Data illustrated in Table 2 show that Licid treatment was more potent to increase serum AST (157% of control animals) followed by Quick treatment (136% of control) and then Newcid treatment (111% of control), while all the tested pediculicides inhibited serum ALT by 31-34%. Regarding the transaminases in livers, data in Table 2 illustrate that the activities of AST decreased by 31, 34, and 32%, while ALT activities decreased by 22, 31 and 28% when mice treated with Newcid, Licid, and Quick, respectively. When the liver cell membrane is damaged, several enzymes such as AST and ALT located in the hepatocyte cytosol are secreted into the blood [81], so they can be used as markers of liver damage [82]. It has been shown that OPs can elevate the enzymatic activities of ALT and AST [83-85]. Therefore, the disruption of transaminases from the normal value denotes biochemical deterioration, and injury of tissues because they are connected with the detoxification process, metabolism, and biosynthesis of active macromolecules for various necessary roles [86]. Also, the increased level of serum AST may be due to increased permeability of the cell membrane or due to cell necrosis and tissue damage.
Effect on ATPase
ATPase is an essential enzyme found in all tissues to hydrolyze phosphate bonds in ATP molecules to form Adenosine Diphosphate (ADP) to release energy [87]. It has essential roles in conserving energy, preventing the cell from swelling, keeping neurons in activity or resting state, transporting the active metabolites, and keeping pH homeostasis [88]. Therefore, the present study aimed to measure the ATPase activity following treatment with the tested pediculicides, which reflects the consumed energy used in the cell [89]. The in vivo effect of the tested pediculicides on some hepatic enzymes in treated and untreated mice are recorded in Table 3. Liver ATPase activities were significantly increased in mice treated with all treatments by 3.11, 2.74, and 1.32-fold control following treatment of mice with Newcid, Licid, and Quick, respectively. The elevation of ATPase activity was recorded after treatment with pyrethroids [90] and OPs [91]. It was documented that Na+ and K+ gradients are related to the secondary transport facilitation for sugar, neurotransmitters, and metabolites; therefore, ATPase is indirectly responsible for the demand of glycogen to glucose conversion. Therefore, malathion, permethrin and tetramethrin found in these commercial pediculicides, like several other insecticides, adversely affect the mitochondrial membrane transport system of hepatocytes [82]. However, several pyrethroids were found to inhibit various ATPases [44] causing non-specific responses such as hepatic and/or renal changes [92,93] associated with neuronal damage caused by generation of excessive reactive oxygen species [94], and defect in mitochondrial bioenergetics, which is commonly observed in neurodegenerative diseases [95].
Effect on ACP
ACP is the lysosomal enzyme playing an important role in catabolism, pathological necrosis, autolysis, phagocytosis, digestion, ion transport, and carbohydrate metabolism [96-99]. Its general reaction mechanism involves hydrolysis of ester phosphate linkages of organophosphate compounds, resulting in the release of inorganic phosphate [100]. Therefore, the cellular membrane damage has been evaluated by measuring the activity of ACP in mice treated with the tested pediculicides. It was found that Licid non-significantly reduced liver ACP activity of mice by 5%, whereas Newcid and Quick significantly reduced liver ACP activity of mice by 7 and 12% of control, respectively (Table 3). Permethrin and cypermethrin were found to significantly inhibit ACP activities in different tissues of mice [92,93]. However, many OPs were found to significantly increase the levels of this enzyme [101,102] and cause a release of some hydrolytic enzymes from lysosomes into the bloodstream [103]. The increment of ACP activity seems to result from enhanced enzyme turnover under pesticide stress, whereas the reduction of its activity may be related to leakage of the enzyme into the extracellular compartment [104,105]. In the present study, because very little inhibition was recorded after pediculicide treatment, therefore ACP activity cannot be utilized as a biomarker for pesticide effects (104).
Table 1: Effect of commercial pediculicides on AChE activity and GABA and glutamic acid levels in mice brain.
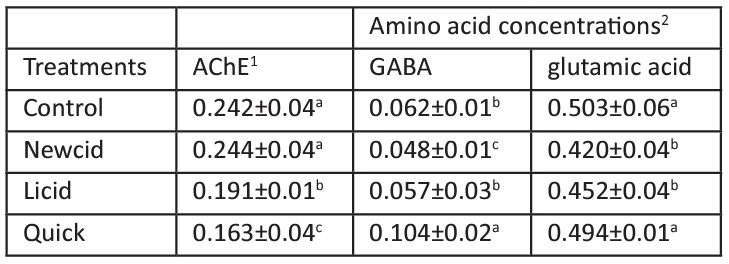
Data are expressed as mean ± S.D (n=5). Means within the same column and having the same letter are not significantly different from each other, p≤ 0.05.
1Specific activity is expressed as mmole of acetylthiocholine hydrolyzed/min/mg protein.
2levels is expressed as mg/mg wet tissue.
Table 2: Effect of commercial pediculicides on transaminases in serum and liver of male mice.
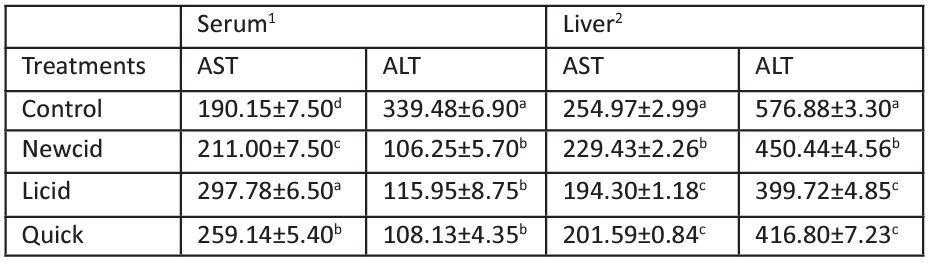
Data are expressed as mean±S.D (n=5). Means within the same column and having the same letter are not significantly different from each other, p≤ 0.05.
1Activity is expressed as units/ml serum.
2Activity is expressed as units/ mg protein of liver.
Table 3: In vivo effect of commercial pediculicides on some hepatic enzymes in male mice.
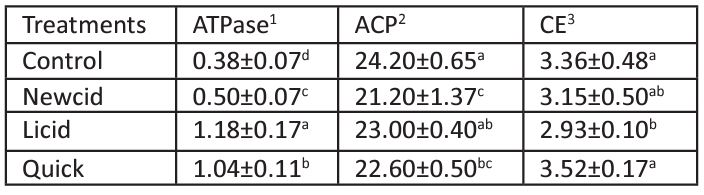
Data are expressed as mean±S.D (n=5). Means within the same column and having the same letter are not significantly different from each other, p≤ 0.05.
1Specific activity is expressed as mmole of inorganic phosphate/min/mg protein.
2Specific activity is expressed as nmole of p-nitrophenol produced/min/mg protein.
3Specific activity is expressed as mmole of p-nitrophenol produced/min/mg protein.
Effect on CarE
Carboxylesterase (CarE) belongs to the superfamily of α/β hydrolase and found in all organisms [106,107] playing a significant role in the metabolism and subsequent detoxification of many agrochemicals by cleaving ester, amide, and thioester linkages [105]. In particular, CarE hydrolyzes pyrethroids [108] and binds to carbamates [109] and OPs to reduce the critical concentration of malaoxon required to inhibit AChE [110,111]. Data presented in Table 3, illustrate that Quick which contains malathion and Newcid which contains permethrin showed non-significant increases and decreases in liver CarE activity, respectively, while Licid contains tetramethrin + PB significantly reduced the activity of liver CarE by 13% of control. CarE has been found to hydrolyze most pyrethroids in mammals to the toxic metabolite, namely 3-phenoxybenzoic acid (3-PBA), the main metabolite, which can enter the dopaminergic neurons to exert the toxic effects such as Parkinson's disease [112], while OPs do not affect mammals although OPs are known as strong anti-CarE [113]. Because this enzyme is part of the metabolic processes and responsible for the degradation of malathion and its active metabolite, malaoxon [27], and the CarE activity in adults and children is lower than in mice [114,115]. Therefore, more care should be considered when these pediculicides are applied to human especially children because children have less mature blood brain barriers and slower rates of clearance [116,117,7].
Conclusion
The present study aimed to investigate the effect of some formulae which are widely used in public health in Egypt as antilice on children on the activities of some biomarkers of mice as experimental animals. It was found that the Licid (0.6% tetramethrin with 2.40% PB) was more toxic than Newcid (1% permethrin), while malathion found in Quick lotion penetrated the skin of treated mice, reached the brain, and significantly inhibited AChE resulted in the disturbance of neurotransmission which is linked with a number of neurological disorders. However, tetramethrin found in Licid formula, which belongs to type I pyrethroids, inhibited only 22% of AChE activity due to the presence of PB. Quick treatment showed higher GABA levels, while Newcid and Licid showed low levels of both GABA and glutamate and increasing or decreasing postsynaptic neuronal activities in the CNS induces neurotoxicity. In the present study, all the pediculicide-treated animals had higher levels of AST levels either in serum or liver ALT compared to control animals. Also, a significant increase in the ATPase level was recorded after all pediculicide treatment and Licid was the most potent to increase the activity, followed by Quick and then Newcid. Products used for the control of lice on children must meet the following requirements: the applied pediculicide must have a sufficiently high toxicity or killing effect to eradicate the lice population, with a minimum level of toxicity and no negative impact to the environment [118]. However, the present study demonstrates that the alteration in different behavioral and biochemical abnormalities represent a high risk for mice following treatments with the lotion and shampoo-based pesticides in mammals. Since there are similarities between different vertebrate groups, disorders observed in mice may indicate risks to children. More investigations should be done to assess the child's risk exposed to such pediculicides. Also, the findings of the present study reinforce the hypothesis already suggested by literature that pediculicide exposure may play a role in children toxicity. Due to the increased toxicity of currently available treatments and the incidence of pediculosis, the search for new, effective products with novel modes of action such as essential oils and herbal products is critical [119]. Thus, it is a reasonable goal to establish significant, safe, healthy and low budget eradication methods to safely eradicate lice infestations.
Statements & Declarations
Funding
The authors declare that no funds, grants, or other support were received during the preparation of this manuscript.
Author contributions
Study concept and design: K.A.O., N.S.A. Analysis: K.A.O., N.S.A, NMA. Statistical analysis: K.A.O. Drafting of the manuscript: K.A.O. Critical revision of the manuscript for important intellectual content: K.A.O.
Data Availability
The data presented in this study are available on request from the corresponding author. The data are not publicly available due to privacy restrictions.
Ethical approval
All animal housing, handling, breeding and bioassays were conducted with the guidelines of the Institutional Animal Care and Use Committee (IACUC), Alexandria University, Egypt.
Conflict of interest
The authors declare that they have no known competing financial interests or personal relationships that could have appeared to influence the work reported in this paper.
Consent to participate
The authors declare that they have no known competing financial interests or personal relationships that could have appeared to influence the work reported in this paper.
Consent to publish
Informed consent was obtained from all individual participants included in the study.
Plant Reproducibility
This article does not contain any studies with plant teproducibility performed by any of the authors.
Clinical trials registration
This study does not contain any studies with clinical trials performed by any of the authors.
Publisher’s note
Springer Nature remains neutral with regard to jurisdictional claims in published maps and institutional affiliations.
References
- Gratz NG. Human lice: Their prevalence, control and resistance to insecticides, World Health Organization, Switzerland. 1997.
- Lee SH, Yoon KS, Williamson MS, Goodson SJ, Takano-Lee M, et al. Molecular analysis of kdr-like resistance in permethrin-resistant strains of head lice, Pediculus capitis. Pestic Biochem Physiol. 2000; 66: 130-143.
- Chosidow O. Scabies and pediculosis. Lancet. 2000; 355: 819-826.
- CDC. Centers for Disease Control and Prevention. November 2, Parasites: Head lice. 2010.
- Liao C-W, Cheng P-C, Chuang TW, ChiuI K-C, Chiang I-C, et al. Prevalence of Pediculus capitis in school children in Battambang, Cambodia. J Microbiol Immunol Infect. 2019; 52: 585-591.
- Mehlhorn H. Blood sucking and chewing lice. In: Rezaei N (ed) Encyclopedia of infection and immunity, Elsevier. 2022; 994-1014.
- Eisenhower C, Farrington EA. Advancements in the treatment of head lice in pediatrics. J Ped Health Care. 2012; 26: 451-461.
- Lebwohl M, Clark L, Levitt J. Therapy for head lice based on life cycle, resistance, and safety considerations. Ped. 2007; 119: 965-974.
- Mumcuoglu KY, Pollack RJ, Reed DL, Barker SC, Gordon S, et al. International recommendations for an effective control of head louse infestations. Inter J Derm. 2021; 60: 272-280.
- Witkowski JA, Parish LC. Pediculosis and Resistance: The Perennial Problem. Clin Dermatol. 2002; 20: 87-92.
- Miyamoto J, Kaneko H, Tsuji R, Okuno Y. Pyrethroids, nerve poisons: how their risks to human health should be assessed. Toxicol Lett. 1995; 82/83: 933-940.
- WHO, World Health Organization. Environmental Health Criteria 94, Permethrin, WHO, Geneva. 1990.
- Tang W, Wang D, Wang J, Wu Z, Li L, et al. Pyrethroid pesticide residues in the global environment: An overview. Chemosphere. 2018; 191: 990-1007.
- Cummings C, Finlay JC, MacDonald NE. Head lice infestations: A clinical update. Ped Child Health. 2018; 23: e18-e24.
- Lawrence LJ, Casida JE. Pyrethroid toxicology: Mouse intracerebral structure-toxicity relationship. Pestic Biochem Physiol. 1982; 18: 9-14.
- Gammon DW, Brown MA, Casida JE. Two classes of pyrethroid action in the cockroach. Pestic Biochem Physiol. 1981; 15: 181-191.
- Narahashi T. Neuronal ion channels as target sites of insecticides. Pharmacol Toxicol. 1996; 78:1-14
- Motomura H, Narahashi T. Interaction of tetramethrin and deltamethrin at the single sodium channel in rat hippocampal neurons. NeuroToxicol. 2001; 22: 329-339.
- Takano-Lee M, Yoon KS, Edman JD, Mullens BA, Clark JM. In vivo and in vitro rearing of Pediculus humanus capitis (Anoplura: Pediculidae). J Med Entomol. 2003; 40: 628-635.
- Andersen HR, David R, Freire C, Fernández MF, D'Cruz SC, et al. Pyrethroids and developmental neurotoxicity - A critical review of epidemiological studies and supporting mechanistic evidence. Environ Res. 2022; 214: 113935.
- Elston DM. Controversies concerning the treatment of lice and scabies. J Am Acad Dermatol. 2002; 46: 794-796.
- Assini FL, Zanette KD, Brocardo PS, Pandolfo P, Rodrigues ALS, et al. Behavioral effects and ChE measures after acute and repeated administration of Malathion in rats. Environ Toxicol Pharmacol. 2005; 20: 443-449.
- Rezg R, Mornagui B, El-Fazaa S, Gharbi N. Biochemical evaluation of hepatic damage in subchronic exposure to Malathion in rats: Effect on superoxide dismutase and catalase activities using native PAGE CR Biologies. 2008a; 331: 655-662.
- Chambers JE. Organophosphorus compounds: An overview, In: Chambers JE, Levi PE (eds) Organophosphorus chemistry, fate and effects, Academic Press, New York. 1992; 3-17.
- Kamath V, Kumar RA, Joshi PS, Rajini PS. Dimethoate induced biochemical perturbations in rat pancreas and its attenuation by cashew nut skin extract. Pestic Biochem Physiol. 2008; 90: 58-65.
- Kamanyire R, Karalliedde L. Organophosphate toxicity and occupational exposure. Occup Med. 2004; 54: 69-75.
- Hazarika A, Sarkar SN, Hajare S, Kataria M, Malik JK. Influence of Malathion pretreatment on the toxicity of anilofos in male rats: a biochemical interaction study. Toxicol. 2003; 185: 1-8.
- Rezg R, Mornagui B, El-Fazaa S, Gharbi N. Caffeic acid attenuates Malathion induced metabolic disruption in rat liver, involvement of acetylcholinesterase activity. Toxicol 2008b; 250: 27-31
- Osman KA, Moretto A, Lotti M. Sulfonyl fluoride and the promotion of diisopropyl fluorophosphate neuropathy. Fund Appl Toxicol. 1996; 33: 294-297.
- Villegas SC, Breitzka RL. Head lice and the use of spinosad. Clin Therap. 2012; 34: 14-23.
- Menegaux F, Baruchel A, Bertrand Y, Lescoeur B, Leverger G, et al. Household exposure to pesticides and risk of childhood acute leukaemia. Occup Environ Med. 2006; 63: 131-134.
- Meinking TL, Taplin D. Safety of permethrin vs. lindane for the treatment of scabies. Arch Dermatol. 1996; 132: 959-962.
- Amanzougaghene N, Fenollar F, Diatta G, Sokhna C, Raoult D, et al. Mutations in GluCl associated with field ivermectin-resistant head lice from Senegal. Intern J Antimicrob Agent. 2018; 52: 593-598.
- Ellman GL, Courtney KK, Andres Jr W, Featherstone RM. A new and rapid colorimetric determination of acetylcholinesterase activity. Biochem. Pharmacol. 1961; 7: 88-95.
- Pepeu G, Bartolnin A, Bartolini R. Differences of GABA content in the cerebral cortex of cats transacted at various midbrain levels. Biochem Pharmacol. 1970; 19: 1007-1013.
- Koch RB. Chlorinated hydrocarbon insecticides: inhibition of rabbit ATPase activities. J Neurochem. 1969; 16: 269-271.
- Reitman S, Frankel S. A colorimetric method for determination of serum glutamic oxaloacetic and glutamic pyruvic transaminases. Am J Clin Pathol. 1957; 29: 56-63.
- Vershoyle RD, Reiner E, Bailley E, Aldridge WN. Dimethyl phosphorothioates. Reaction with malathion and effect on malathion toxicity. Arch Toxicol. 1982; 49: 293-301.
- Bessy DA, Lowry OH, Broch MI. A method for the rapid determination of alkaline phosphatase with five cubic millimeters of serum. J Biol Chem. 1946; 164: 321-329.
- Lowry OH, Rosebrough NJ, Farr AL, Randal RJ. Protein measurement with folin phenol reagent. J Biol Chem. 1951; 193: 265-275.
- Costat Program. Version 2, Cohort Software, Minneapolis, MN, USA. 1986.
- Durand R, Bouvresse S, Berdjane Z, Izri A, Chosidow O, et al. Insecticide resistance in head lice: Clinical, parasitological and genetic aspects. Clin Microbiol Infect Off Pub Eur Soc Clin Microbiol Infect Dis. 2012; 18: 338-344.
- Anadón A, Martínez M, Martínez MA, Díaz MJ, Martínez-Larrañaga MR. Toxicokinetics of lambda-cyhalotrin in rats. Toxicol Lett. 2006; 165: 47-56.
- Narahashi T. Nerve membrane ionic channels as the primary target of pyrethroids. Neuro Toxicol. 1985; 6: 3-22.
- Vijverberg HPM, van den Bercken J. Neurotoxicological effects and mode of action of pyrethroid insecticides. Crit Rev Toxicol. 1990; 21: 105-126.
- Wilks MF. Pyrethroid-induced paresthesias: A central or local toxic effect? Clin Toxicol. 2000; 38: 103-105.
- Orion E, Matz H, Ruocco V, Wolf R. Parasitic skin infestations II, scabies, pediculosis, spider bites: Unapproved treatments. Clin Dermatol. 2002; 20: 618-625.
- Naeher LP, Barr DB, Rithmire N, Edwards J, Holmes AK, et al. Pesticide exposure resulting from treatment of lice infestation in school-aged children in Georgia. Environ Inter. 2009; 35: 358-362.
- ATSDR. The Agency for Toxic Substances and Disease Registry. Toxicological profile for pyrethrins and pyrethroids. Atlanta: Agency for Toxic Substances and Disease Registry. 2003.
- Nash B. Treating head lice. BMJ. 2003; 326: 1256-1257.
- Tomlin CDS. The e-Pesticide Manual, Version 2.2. The British Crop Protection Council, Surrey, UK. 2002.
- Kaur G, Arora SK. Acetylcholinesterase and Na+, K+-ATPase activities in different regions of rat brain during insulin-induced hypoglycemia. Mol Chem Neuropathol. 1994; 21: 83-93.
- Lionetto MG, Caricato R, Calisi A, Giordano ME, Schettino T. Acetylcholinesterase as a biomarker in environmental and occupational medicine: new insights and future perspectives. Biomd Res Inter. 2013; 1: 1-8.
- Osman KA, Shaaban MMI, Ahmed NS. Biomarkers of imidacloprid toxicity in Japanese quail, Coturnix coturnix Japonica. Environ. Science and Pollut. Res. 2022.
- Moores GD, Philippou D, Borzatta V, Trincia P, Jewess P, et al. An analogue of piperonyl butoxide facilitates the characterisation of metabolic resistance. Pest Manag Sci. 2009; 65: 150-154.
- Horton MK, Rundle A, Camann DE, Boyd Barr D, Rauh VA, et al. Impact of prenatal exposure to piperonyl butoxide and permethrin on 36-month neurodevelopment. Ped. 2011; 127: e699-706.
- Gaire S, Zheng W, Scharf MF, Gondhalekar AD. Plant essential oil constituents enhance deltamethrin toxicity in a resistant population of bed bugs (Cimex lectularius L.) by inhibiting cytochrome P450 enzymes. Pestic Biochem Physiol. 2021; 175: 104829.
- Husain R, Husain R, Adhami VM, Seth PK. Behavioral, neurochemical, and neuromorphological effects of deltamethrin in adult rats. J Toxicol Environ Health. 1996; 48: 515-526.
- Hossain MM, Suzuki T, Sato I, Takewaki T, Suzuki K, et al. The modulatory effect of pyrethroids on acetylcholine release in the hippocampus of freely moving rats. NeuroToxicol. 2004; 25: 825-833.
- Hossain MM, Tadahiko Suzuki T, Sato I, Takewaki T, Suzuki K, et al. Neuromechanical effects of pyrethroids, allethrin, cyhalothrin and deltamethrin on the cholinergic processes in rat brain. Life Sci. 2005; 77: 795-807.
- Taplin D, Castillero PM, Spiege J, Mercer S, Rivera AA, et al. Malathion for treatment of Pediculus humanus var capitis infestation. JAMA. 1982; 247: 3103-3105.
- Yang YC, Choi HY, Choi WS, Clark JM, Ahn YJ. Ovicidal and adulticidal activity of Eucalyptus globulus leaf oil terpenoids against Pediculus humanus capitis (Anoplura: Pediculidae). J Agric Food Chem. 2004; 52: 2507-2511.
- Enan E (2001) Insecticidal activity of essential oils: octopaminergicsites of action. Comp Biochem Physiol Part C: Toxicol Pharmacol. 2001; 130: 325-337.
- Mills C, Cleary BJ, Gilmer JF, Walsh JJ. Inhibition of acetylcholinesterase by tea tree oil. J Pharm Pharmacol. 2004; 56: 375-379.
- Aldridge WN, Miles JW, Mount DL, Verschoyle RD. The toxicological properties of impurities in malathion. Arch Toxicol. 1979; 42: 95-106.
- Buratti FM, Testai E. Malathion detoxification by human hepatic carboxylesterases and its inhibition by isomalathion and other pesticides. J Biochem Mol Toxicol. 2005; 19: 406-414.
- Dennis GA, Lee PN. A phase I volunteer study to establish the degree of absorption and effect on cholinesterase activity of four head lice preparations containing malathion. Clin Drug Invest. 1999; 18: 105-115.
- Robinson MB, Coyle JT. Glutamate and related acidic excitatory neurotransmitters: From basic science to clinical application. FASEB J. 1987; 1: 446-454.
- Cooper JR, Bloom FE, Roth RH. Amino acid transmitters. In: The biochemical basis of neuropharmacology, 8th edn. Oxford, NY, Oxford University Press. 2003; 105-150.
- Pitzer EM, Williams MT, Vorhees CV. Effects of pyrethroids on brain development and behavior: Deltamethrin. Neurotoxicol Terat. 2021; 87:106983.
- Kullmann DM, Asztely F, Walker MC. The role of mammalian ionotropic receptors in synaptic plasticity: LTP, LTD and epilepsy. Cell Mol Life Sci. 2000; 57: 1551-1561.
- Bloomquist JR, Adams PM, Soderlund DM. Inhibition of gamma-aminobutyric acid-stimulated chloride flux in mouse brain vesicles by polychloroalkane and pyrethroid insecticides. Neurotoxicol. 2008; 7: 11-20.
- Hossain MM, Suzuki T, Unno T, Komori S, Kobayashi H. Differential presynaptic actions of pyrethroid insecticides on glutamatergic and GABAergic neurons in the hippocampus. Toxicol. 2008; 243: 155-163.
- Bist R, Bhatt DK. The evaluation of effect of alpha-lipoic acid and vitamin E on the lipid peroxidation, gamma-amino butyric acid and serotonin level in the brain of mice (Mus musculus) acutely intoxicated with lindane. J Neurol Sci. 2009; 276: 99-102.
- Eells JT, Dubocovich ML (1988) Pyrethroid insecticides evoke neurotransmitter release from rabbit striatal slices. J Pharmacol Exp Ther. 1988; 246: 514-521.
- Kirby ML, Castagnoli K, Bloomquist JR. In vivo effects of deltamethrin on dopamine neurochemistry and the role of augmented neurotransmitter release. Pestic Biochem Physiol. 1999; 65: 160-168.
- Wang X, Wu J, Xiao H, Shi Y. Effects of fenvalerate and phoxim insecticides on glutamate and gamma-aminobutyric acid immunoreactive cells in the central nervous system of rats. Wei Sheng Yan Jiu. 2001; 29: 1-3.
- Lopatina OL, Komleva YK, Gorina YV, Olovyannikova RY, Trufanova LV, et al. Oxytocin and excitation/inhibition balance in social recognition. Neuropep. 2018; 72: 1-11.
- Lanter LA. "Aminotransferases" clinical biochemistry, Saunders WB (ed), (7th edn) Comp, Philadelphia, London, Toronto. 1978; 553.
- Coremen M, Turkyilmaz IB, Us H, Us AS, Celik S, et al. Lupeol inhibits pesticides induced hepatotoxicity via reducing oxidative stress and inflammatory markers in rats. Food Chem Toxicol. 2022; 164: 113068.
- Ncibi S, Othman MB, Akacha A, Krifi MN, Zourgi L. Opuntia ficus indica extract protects against chlorpyrifos-induced damage on mice liver. Food Chem Toxicol. 2008; 46: 797-802.
- Gokcimen A, Gulle K, Demirin H, Bayram D, Kocak A, et al. Effects of diazinon at different doses on rat liver and pancreas tissues. Pestic Biochem Phys. 2007; 87:103-10.
- Campbell PI, OO Ofurum. Serum and liver enzyme changes in rats after short term exposure to dichlorvos. Comp Biochem Physiol C. 1986; 83: 443-446.
- Celik I, Yilmaz Z, Turkoglu V. Hematotoxic and hepatotoxic effects of dichlorvos at sublethal dosages in rats. Environ Toxicol. 2009; 24: 128-132.
- Kalender S, Uzun FG, Durak D, Demir F, Kalender Y. Malathion-induced hepatotoxicity in rats: The effects of vitamins C and E. Food Chem Toxicol. 2010; 48: 633-638.
- Ambali S, Akanbi D, Igbokwe N, Shittu M, Kawu M, et al. Evaluation of subchronic chlorpyrifos poisoning on hematological and serum biochemical changes in mice and protective effect of vitamin C. J Toxicol Sci. 2007; 32: 111-120.
- Stewart AG, Laming EM, Sobti M, Stock D. Rotary ATPases dynamic molecular machines. Curr Opin Struct Biol. 2014; 25: 40-48.
- Chakraborti S, Dhalla NS (eds). Regulation of membrane Na+- K+ ATPase. Springer International Publishing. 2016.
- Hai CM, Murphy RA. Cross-bridge phosphorylation and regulation of latch state in smooth muscle. Amer J Phys-Cell Phys. 1988; 254: C99-C106.
- Jones OT, Lee AG. Effect of pyrethroids on the activity of purified (Ca2+/Mg2+)-ATPase. Pestic Biochem. 1986; 25: 420-430.
- Osman KA. Interaction of glyphosate with some mammalian biochemical targets. Alex J Agric Res, Egypt. 1994; 39: 373-392.
- Osman KA, Salama AK. Enzyme alteration following a single or repeated doses of cypermethrin in mice. Comm Sci Dev Res, Egypt. 1994; 48: 95-112.
- Salama AK, Osman KA. Comparative studies of enzyme modulation following permethrin administration in mice. Com Sci Dev Res. 1994; 48: 201-219.
- Uttara B, Singh AV, Zamboni P, Mahajan RT. Oxidative stress and neurodegenerative diseases: A review of upstream and downstream antioxidant therapeutic options. Curr Neuropharmacol. 2009; 7: 65-74.
- Kinoshita PF, Leite JA, Orellana AMM, Vasconcelos AR, Quintas LEM, et al. The influence of Na+, K+-ATPase on glutamate signaling in neurodegenerative diseases and senescence. Front Physiol. 2016; 7: 195.
- Cheung WWK, Low KW. Ultrastructural and functional differentiation of the midgut of the sugar cane beetle, Protaetia acuminata (F.) (Coleoptera: Cetoniidae). Int J Insect Morphol Embryol. 1975; 4: 349-361.
- Abou-Donia MB. Increased acid phosphatase activity in hens following an oral dose of Leptophos. Toxicol Lett. 1978; 2: 199-203.
- Yi S-X, Adams TS. Age- and diapause-related acid and alkaline phosphatase activities in the intestine and malpighian tubules of the Colorado potato beetle, Leptinotarsa decemlineata (Say). Arch Insect Biochem Physiol. 2001; 46:152-163.
- Sreenivasan RS, Krishna Moorthy P, Deecaraman M. Cypermethrin induced toxicity to phosphatases and dehydrogenases in gills and hemolymph of fresh water crab, Spiralothelphusa hydrodroma (herbst). Int J Biol Med Res. 2011; 2: 784-788.
- Hoehamer CF, Mazur CS, Wolfe NL. Purification and partial characterization of an acid phosphatase from Spirodela oligorrhiza and its affinity for selected organophosphate pesticides. J Agric Food Chem. 2005; 53: 90-97.
- Abou-Donia MB, Abdo KM, Timmonsand PR, Proctor JE. Brain acetylcholinesterase, acid phosphatase, and 2’, 3’-cyclic nucleotide-3’-phosphohydrolase with plasma butyrylcholinesterase activities in hens treated with a single dermal neurotoxic dose of S,S,S-tri-nbutyl phosphorotrithioate. Toxicol Appl Pharmacol. 1986; 82:461-473.
- Kumari K, Sinha RC. Biochemical changes in the toad, Bufo melanostictus as a function of methyl parathion: ascorbic acid as a biomarker of oxidative stress. Вісник Харківського національного університету імені ВН Каразіна. Серія: Біологія. 2010; 12: 90-97.
- Lozano-Paniagua D, Parron T, Alarcon R, Requena M, Lopez-Guarnido O, et al. Evaluation of conventional and non-conventional biomarkers of liver toxicity in greenhouse workers occupationally exposed to pesticides. Food Chem Toxicol. 2021; 151: 112127.
- Mazzei F, Botre F, Botre C. Acid phosphatase/glucose oxidase-based biosensors for the determination of pesticides. Anal Chem Acta. 1996; 336: 67-75.
- Redinbo MR, Potter PM. Mammalian carboxylesterases: from drug targets to protein therapeutics. Drug Discov Today. 2005; 10: 313-325.
- Wheelock CE, Shan G, Ottea J. Overview of carboxylesterases and their role in the metabolism of insecticides. J Pestic Sci. 2005; 30: 75-83.
- Li Y, Sun H, Tian Z, Li Y, Ye X, et al. Identification of key residues of carboxylesterase PxEst-6 involved in pyrethroid metabolism in Plutella xylostella (L.). J Hazard Mat. 2021; 407: 124612.
- Wheelock CE, Miller JL, Miller MG, Shan G, Gee SJ, et al. Development of Toxicity Identification Evaluation (TIE) procedures for pyrethroid detection using esterase activity. Environ Toxicol Chem. 2004; 23: 2699-2708.
- Sogorb MA, Vilanova E. Enzymes involved in the detoxification of organophosphorus, carbamate and pyrethroid insecticides through hydrolysis. Toxicol Lett. 2002; 128: 215-228.
- Karanth S, Olivier Jr K, Liu J, Pope C. In vivo interaction between chlorpyrifos and parathion in adult rats: Sequence of administration can markedly influence toxic outcome. Toxicol Appl Pharmacol. 2022; 177:247-255.
- Casida JE, Quistad GB. Organophosphate toxicology: safety aspects of nonacetylcholinesterase secondary targets. Chem Res Toxicol. 2004; 17: 983-998.
- Wan F, Yu T, Hu J, Yin S, Li Y, et al. The pyrethroids metabolite 3-phenoxybenzoic acid induces dopaminergic degeneration. Sci Total Environ. 2022; 838: 156027.
- Stenersen J (2004) Chemical pesticides. Mode of action and toxicology. CRC Press, Boca Raton
- Kaliste-Korhonen E, Touvinen K, Hanninen O. Interspecies differences in enzymes reacting with organophosphates and their inhibition by paraoxon in vitro. Hum Exp Toxicol. 1996; 15: 972-978.
- Mallick P, Moreau M, Song G, Efremenko AY, Pendse SN, et al. Development and application of a life-stage Physiologically Based Pharmacokinetic (PBPK) model to the assessment of internal dose of pyrethroids in humans. Toxicol Sci. 2022; 173: 86-99.
- Mortuza T, Chen C, White CA, Cummings BS, Muralidhara S, et al. Toxicokinetics of deltamethrin: Dosage dependency, vehicle effects, and low-dose age-equivalent dosimetry in rats Toxicol Sci. 2018; 162: 327-336.
- Allegaert K, van den Anker J. Ontogeny of phase I metabolism of drugs. J Clin Pharmacol. 2019; 59: S33-S41.
- Ten Bosch L, Habedank B, Siebert D, Mrotzek J, Viöl W. Cold atmospheric pressure plasma comb- a physical approach for pediculosis treatment. Int J Environ Res. Public Health. 2019; 16: 1-16.
- Soonwera M, Wongnet O, Sittichok S. Ovicidal effect of essential oils from Zingiberaceae plants and Eucalytus globuluson eggs of head lice, Pediculus humanus capitis De Geer, Phytomed. 2019; 47: 93-104.