Case Report - Volume 3 - Issue 5
Systemic air embolism after percutaneous lung puncture: A case report
Jing-Si Wu, MM¶; Hong-Jiao Xu, MM¶; Jie Qi, Ph.D, MD; Peng-Xia Zhu, MM; Zhao-Min Wang,MD; Ya-Chun Zhou, Ph.D, MD; Yu-Yu Gu, BD; Min-Min Zhu, Ph.D, MD; Jin-Bao Li, Ph.D, MD
Department of Anesthesiology, Shanghai Jiaotong University First People’s Hospital (Shanghai General Hospital), Shanghai, China.
¶These authors are co-first authors and contributed equally to this study
Received Date : August 18, 2023
Accepted Date : Sep 15, 2023
Published Date: Sep 22, 2023
Copyright: © Jin-Bao Li and Min-MinZhu 2023
*Corresponding Author: Jin-Bao Li and Min-Min Zhu, Department of Anesthesiology, Shanghai Jiaotong University First People’s Hospital (Shanghai General Hospital), Shanghai, China.
Email: zhu_mm@126.com
DOI: Doi.org/10.55920/2771-019X/1553
Abstract
Background: Systemic air embolism (SAE) is a rare but potentially fatal complication that occurs during percutaneous lung puncture. There is limited data on the diagnosis and treatment of SAE. Here, we reported a case of systemic air embolism during presurgical localization of thoracoscopic pulmonary nodule resection and documented the frequent presence of air in the heart chambers.
Case presentation: We describe a case of systemic air embolism after percutaneous lung puncture for the presurgical localization of thoracoscopic pulmonary nodule resection. The patient was a 75-year-old male, ethnic Han. The primary finding was sudden cardiac dysfunction, decreased invasive arterial pressure along with a sudden decreased heart rate and decreased pulse oxygen saturation. With immediate and effective cardiopulmonary resuscitation and a high dose of epinephrine, the patient recovered with stable vital signs and exhibited transient myocardial ischemia. Echocardiography documented the presence of air in the heart chambers. The occurrence time of the seizure was 24 hours after surgery. Intravenous sodium valproate and midazolam therapy was administered. A right cerebral cortex injury appeared 3 days later, and the patient was conscious with left hemiplegia. Seven days later, he answered fluently and perceptually, his left upper limb moved freely, and his left lower limb muscle strength turned from grade Ⅱ to Ⅲ. Then, the patient was discharged from the hospital and transferred to a rehabilitation hospital.
Conclusions: The occurrence of SAE is quick and the complication is deadly. Combination of electrocardiography (ECG) and early echocardiography for the identification of SAE. Arterial pressure, end tidal CO2 (ETCO2), and other vital life parameters were monitored in a timely manner. Prompt emergency resuscitation with chest compressions and I.V. epinephrine can prevent morbidity and mortality. Early rehabilitation therapy is effective in improving the outcomes of patients.
Keywords: Air embolism; Lung puncture; Lung cancer; Cardiopulmonary resuscitation; Myocardial ischemia; Cerebral cortex
Abbreviations:
SAE- Systemic Air Embolism
L-DLT- Left Double Lumen Tube
ECG- Electrocardiographic
ETCO2- End Tidal CO2
VF- Ventricular Fibrillation
IPPV/O2- Intermittent Positive-Pressure Oxygen Ventilation
MI- Myocardial Infraction
CK-MB - Creatine Kinase MB
MRI - Magnetic Resonance Imaging
CT - Computed Tomography
Introduction
The incidence of systemic air embolism (SAE) during percutaneous lung puncture is between 0.02% and 0.21%, and the occurrence is rare but potentially fatal [1-3]. During percutaneous lung puncture with positive pressure ventilation, air passes into the pulmonary vein if alveolar or bronchial pressure is higher than venous pressure, thus forming SAE. Coronary and cerebral embolism lead to cardiac and neurologic ischemia [3, 4]. Several diagnostic tools can detect intracardiac and cerebral embolism, such as TEE, Doppler and CT [5, 6]. Small amounts of air embolism may cause cardiac or neurological symptoms depending on the affected arteries, and large amounts of air embolism can lead to fatal cardiovascular collapse [7]. Although there are various systematic reviews and meta-analyses on air embolism, we reported a case of SAE with sudden cardiac dysfunction and documented the frequent presence of air bubbles in the heart chambers in the operation room, and presented the prognosis of it. Along with chest compressions, and I.V. epinephrine, the patient’s hemodynamic parameters were normal, and 7 days later, his limb movement disorder spontaneously recovered. Therefore, anesthetists must remain vigilant with SAE during thoracic surgery. Here, we describe a case of SAE after percutaneous lung puncture for the presurgical localization of thoracoscopic pulmonary nodule resection and share our rescue experience. We provided ideals of quick rescue and recovery in the face of SAE, and we successfully brought the patient’s life signs back. These discussions fill the gaps among other researchers’ studies.
Case presentation
A 75-year-old male patient with bilateral pulmonary nodules eight months after colon surgery was admitted for thoracoscopic pulmonary nodule resection. The patient was anesthetized and intubated with a left double lumen tube (L-DLT). After that, the artery was catheterized for continuous invasive blood pressure. Correct ideal anatomic placement of the L-DLT was ensured by the visual screen, and positive-pressure ventilation with oxygen 100% was performed. The initial ETCO2 value was 35 mmHg, heart rate was 87 beats per minute (bpm), SPO2 was 100%, and invasive arterial pressure was 137/78 mmHg. To locate the pulmonary nodules for thoracoscopic resection, a percutaneous lung puncture was performed at the right lateral position with positive pressure ventilation. A 20-gauge needle is placed percutaneously through the chest wall into the pulmonary nodule. Then, 0.05 ml of diluted methylene blue was injected through the localizing needle to stain the subpleural area overlying the nodule. The puncture point was bandaged. Within 2 minutes after the puncture, the multiparameter life sign monitor displayed ECG changes, including supraventricular tachycardia and frequent ventricular premature beats. Invasive arterial pressure (85/40 mmHg) and heart rate (85 bpm) dropped, pulse oxygen saturation suddenly decreased to 83%, and ETCO2 dropped to 22 mmHg (Fig. 1). We quickly changed the position from lateral to supine and injected adrenalin (0.05 mg) intravenously. Then, the ECG showed new ventricular fibrillation (VF), one type of arrhythmia that is deadly. Both pulse oxygen saturation and ETCO2 could not be measured (Fig. 1). An external defibrillation was administered promptly. Emergency resuscitation was performed immediately by intermittent positive-pressure oxygen ventilation (IPPV/O2), chest compressions and I.V. epinephrine (2 mg) and 5% sodium bicarbonate (50 ml). Three minutes later, the life sign monitor showed elevated arterial blood pressure (168/115 mmHg) and increases in pulse oxygen saturation (100%) and ETCO2 (35 mmHg), indicating that the patient’s hemodynamic parameters were normal. Then we transferred the patient to hyperbaric chamber. Thirty minutes later, the ETCO2 value was 35 mmHg, heart rate was 94 bpm, SPO2 was 100%, and blood pressure was 103/62 mmHg (Fig. 1). The patient’s preoperative ECG was normal (Fig. 2 a). After life signs returned, bedside ECG findings showed sinus tachycardia, acute inferior wall myocardial infarction and right bundle branch block (Fig. 2 b), which indicated myocardial infarction (MI). One hour later, ECG showed sinus rhythm and ST-segment changes (Fig. 2 c). Three hours later, ECG findings exhibited sinus rhythm, T-wave changes and pathologic Q waves (Fig. 2 d). These data indicated that transient ischemia occurred in cardiac muscle. Patients with transient myocardial ischemia have a relatively high risk of MI. Right after resuscitation, echocardiography documented the frequent presence of air in the heart chambers (Fig. 3). Six hours later, two-dimensional and pulsed-wave Doppler echocardiography showed left ventricular regional systolic dysfunction, which may be
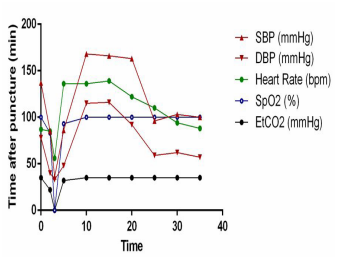
Figure 1: Invasive arterial pressure gradually decreased to 36/33 mmHg, pulse oxygen saturation decreased to 83%, and ETCO2 dropped to 22 mmHg. Three minutes later, the life sign monitor showed elevated arterial blood pressure and a rise in pulse oxygen saturation and ETCO2, and the patient’s hemodynamic parameters were normal.
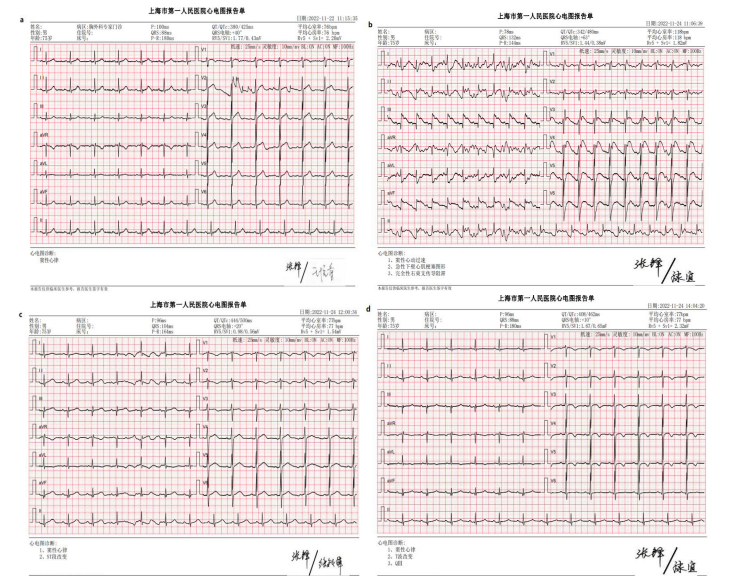
Figure 2: Preoperative ECG was normal (a). ECG findings showed sinus tachycardia, acute inferior wall myocardial infarction and right bundle branch block (b). One hour later, ECG showed sinus rhythm and ST-segment changes (c). Three hours later, ECG findings exhibited sinus rhythm, T-wave changes and pathologic Q wave (d).
the sequelae of MI. The cardiac-specific serum marker myoglobin (MB) content value was 252 ng/ml (normal value: 0-70 ng/ml). Two hours later, the MB content value fell to 78.4 ng/ml. Ten hours later, the MB content value was 43.7 ng/ml (Fig. 4 a). The cardiac troponins allow us to track the extent of injury suffered from the myocardium. The troponin content value was 0.185 ng/ml (normal value: 0-0.04 ng/ml). Two hours later, the troponin content value rose to 5.67 ng/ml. Ten hours later, the troponin content value was 2.9 ng/ml (Fig. 4 b). The serum marker of acute myocardial infarction creatine kinase MB (CK-MB) content value was 3.9 ng/ml (normal value: 0-4 ng/ml). Two hours later, the CK-MB content value was 33.4 ng/ml. Ten hours later, the CK-MB content value was 25 ng/ml, maintaining a high value compared to the normal value (Fig. 4 c). The typical rise and fall of serum levels of biochemical markers remains a cornerstone in the diagnosis of MI.
The patient remained apathetic and had involuntary limb movement. Seizure occurred at 24 hours after surgery. Intravenous sodium valproate (200 mg) and midazolam (2 mg) therapy was administered. Brain magnetic resonance imaging (MRI) analysis showed damage in the cerebral cortex, while brain computed tomography (CT) scan was normal. Intravenous antibiotic therapy with cefoxitin was initiated for suspected sepsis.
At a 3-day follow-up, the patient was conscious, and his right limb moved freely, but the left limb lacked muscle afferent feedback. Five days later, the patient started enteral nutrition. Rehabilitation training for motor recovery was carried out. Seven days later, his left upper limb could move freely, and his left lower limb muscle strength turned from grade Ⅱ to Ⅲ. Then, he was discharged from the hospital and transferred to a rehabilitation hospital. Urine cultures taken during hospitalization failed to grow pathogenic organisms.
Discussion
SAE is a rare but potentially fatal condition that occurs when atmospheric air enters the pulmonary vein during lung biopsy, serious chest trauma, arterial catheterization or cardiopulmonary bypass [5]. Air embolizes from the pulmonary venous system mainly to coronary and cerebral arteries. A total of 0.5-1
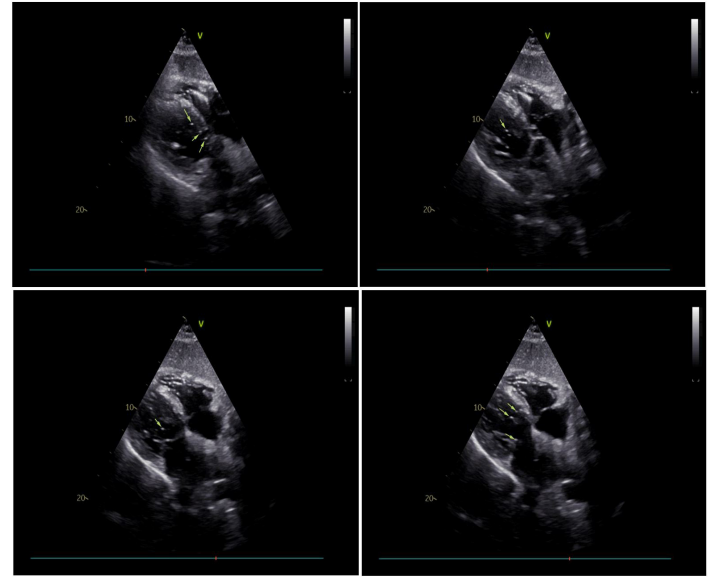
Figure 3: Echocardiography documented the frequent presence of air in the heart chambers.
ml of air injected into a pulmonary vein can cause cardiac arrest from coronary air embolism and ischemia, and 2 mL of air injected into cerebral circulation can be fatal [8, 9]; thus, high airway pressures must be avoided to reduce air intake. The risk factors for SAE were the presence of an underlying disease, the use of a ≥19-gauge needle, and coronary or intracranial air embolism [10]. When there is direct communication between air and damaged veins along with pressure, the air transfers into the circulation [11]. The classic findings are hemoptysis and sudden cardiac or cerebral dysfunction [8]. The patient described in the present report met these criteria by receiving a percutaneous lung puncture and positive pressure ventilation. Moreover, this patient exhibited echocardiography images documenting the existence of air embolism in heart chambers in quantity and symptoms compatible with SAE [12].
During the progress of lung puncture, there are three ways that air can enter the pulmonary venous system. First, the needle can penetrate the pulmonary vein, which can create a fistula between the air-containing space (for example, the pulmonary alveolar space) and the pulmonary vein. Positive-pressure ventilation can increase the pressure in the air-containing space, which results in air embolism. Second, when the needle is placed in the pulmonary vein and then removed, the pulmonary vein is directly exposed to the atmosphere, and air may enter the pulmonary vein through the aisle when atmospheric pressure exceeds the pulmonary vein pressure, as mechanical ventilation can decrease cardiac output and pulmonary vein pressure. Third, air may enter pulmonary arterial circulation and then reach veins through the microvasculature [13, 14]. In our study, air entered the vein system in the first way.
One risk factor for SAE was the presence of an underlying disease. The presence of systemic medical illness can worsen a patient’s serious condition [10]. For example, there is an increased risk of cardiovascular disease and a dismal prognosis after acute coronary events when diabetes is present, and nonalcoholic fatty liver disease (NAFLD) accelerates arteriosclerosis, thereby increasing cardiovascular risk and associated cardiovascular events, as it can heighten the systemic proinflammatory state [15-18]. Therefore, we need to know the underlying diseases and their influence on deadly emergency diseases to develop good treatment options.
A noteworthy aspect of this case was the patient’s fatal response to the acute SAE event. Invasive arterial pressure decreased, and noninvasive pressure could not be measured.
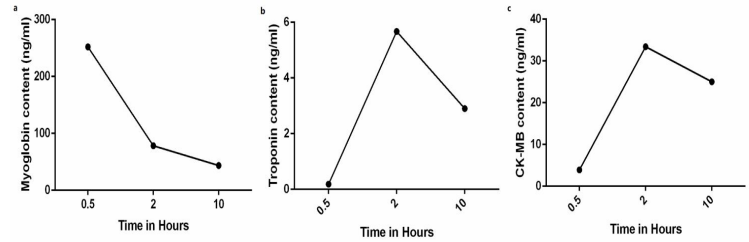
Figure 4: The MB content value was 252 ng/ml. Two hours later, the MB content value fell to 78.4 ng/ml. Ten hours later, the MB content value was 43.7 ng/ml (a). The troponin content value was 0.185 ng/ml. Two hours later, the troponin content value rose to 5.67 ng/ml. Ten hours later, the troponin content value was 2.9 ng/ml (b). The CK-MB content value was 3.9 ng/ml. Two hours later, the CK-MB content value was 33.4 ng/ml. Ten hours later, the CK-MB content value was 25 ng/ml (c).
When gas bubbles are introduced into the blood stream and then into the coronary artery, these bubbles may cause the cessation of blood flow within the coronary artery. The heart and brain are vulnerable to short ischemia, and oxygen starvation due to air embolism in the coronary artery can cause cellular damage and irreversible injury. Thus, systolic function of the left ventricle is impaired [19]. Arterial pressure is a key hemodynamic variable. Moreover, intra-arterial catheters and invasive blood pressure monitoring are considered the “gold standard” for arterial pressure monitoring. Critically ill patients and patients undergoing high-risk and major surgery are supposed to be instrumented with invasive blood pressure [20].
During the event, the patient’s pulse oxygen saturation decreased, and ETCO2 dropped suddenly. ETCO2 is a quantitative measurement of cardiac arrest, and ETCO2 decreased by 23%±8% from pre-VF to during VF [21]. Decreased ETCO2 is related to decreased pulmonary blood flow and decreased cardiac output. Animal studies have suggested that ETCO2 correlates with coronary artery perfusion and perhaps cerebral perfusion pressure [22]. CO2 produced by tissue aerobic metabolism diffuses from cells into the blood capillary, is transported by veins to the lung, and is removed by ventilation. The determinants include tissue aerobic metabolism, cardiac output, lung perfusion and alveolar ventilation. When there is ventilation/perfusion mismatch in the lung due to pulmonary embolism or lung hypoperfusion during cardiac arrest, the ETCO2 value decreases. ETCO2 measurements during cardiac arrest predict successful resuscitation. Capnography is a noninvasive estimate of cardiac output and organ perfusion during cardiac arrest and can be used to monitor the quality of CPR [23].
Coronary air emboli induce transient cardiac ischemia [8], early chest compression and I.V. epinephrine helps to maintain blood pressure and blood supply to vital organs. Prompt clinical and radiologic recognition of SAE can significantly prevent morbidity and mortality. In this case, the inspired air was 100% oxygen; thus, the main component of the embolism was oxygen, which was easily absorbed. This may explain this patient’s good prognosis. Hyperbaric chamber is a priority and the transport to the facility should immediately be organized. Hyperbaric oxygen therapy is recommended because it may facilitate gas reabsorption to decrease the size of air emboli, and it can also improve tissue oxygenation and relieve tissue ischemic reperfusion injury [5]. Anesthetics consistently and meaningfully improve outcomes because of their early neuroprotection after cerebral ischemia. Drugs selected during anesthesia for surgery suppress neurotransmission and reduce energy consumption, which allows tissue to better preserve energy balance during ischemic injury. Appropriate use of drugs such as propofol, etomidate and lidocaine increases tolerance to ischemia, while factors including brain temperature, blood glucose, and perfusion are important determinants of ischemia outcomes. Deep hypothermia (18-22 ℃) is highly neuroprotective. The regulation of blood glucose yields good outcomes in stroke patients. Patients with worse outcomes from cerebral ischemia tend to have higher blood glucose concentrations [24].
Conclusions
In conclusion, percutaneous lung puncture with positive pressure ventilation favors air entry into the pulmonary vein, and coronary air emboli induces transient cardiac ischemia and VF, one type of fatal arrhythmia. Monitoring arterial pressure, ETCO2 and other vital life parameters in a timely manner. Prompt emergency resuscitation with chest compressions and I.V. epinephrine can prevent morbidity and mortality. Early rehabilitation therapy is effective in improving the outcomes of patients.
References
- MC Freund, J Petersen, KC Goder, et al. Systemic air embolism during percutaneous core needle biopsy of the lung: frequency and risk factors. BMC pulmonary medicine, 2012, 12(1): 1-12.
- H Ishii, T Hiraki, H Gobara, et al. Risk factors for systemic air embolism as a complication of percutaneous CT-guided lung biopsy: multicenter case-control study. Cardiovascular and interventional radiology. 2014; 37(5): 1312-1320.
- V Arora, G Burks. Systemic Air Embolism during Percutaneous Transthoracic Lung Biopsy. Anesthesiology. 2021; 135(6): 1120-1120.
- QQ Dai, WH Liu, Y Li, et al. A Successfully Resuscitated Case of Air Embolism during Vitrectomy. Chinese Medical Journal, 2018, 131(12): 1509-1510.
- N Malik, PL Claus, JE Illman, et al. Air embolism: diagnosis and management. Future Cardiology. 2017; 13(4): 365-378.
- HJ Huang, S Lei, L Yang, et al. Systemic air embolism in a fungal pneumonia patient with lung cavities formation and review of literature. Chinese Journal of Traumatology. 2019; 22(5): 308-310.
- M Kramer, M Parekh, RY Modi, et al. Review of thoracic causes of systemic arterial air embolism on computed tomography. Journal of Thoracic Imaging. 2020; 35(3): W68-W74.
- Anthony MH, Ho E. Ling. Systemic Air Embolism after Lung Trauma . Anesthesiology. 1999; 90(2): 564-575.
- C Sun, J Bian, S Lai, et al. Systemic air embolism as a complication of CT-guided percutaneous core needle lung biopsy: a case report and review of the literature. Experimental and Therapeutic Medicine. 2015; 10(3): 1157-1160.
- JH Lee, SH Yoon, H Hong, et al. Incidence, risk factors, and prognostic indicators of symptomatic air embolism after percutaneous transthoracic lung biopsy: a systematic review and pooled analysis. European Radiology. 2021; 31: 2022-2033.
- T Kapoor, G Gutierrez. Air embolism as a cause of the systemic inflammatory response syndrome: a case report. Crit Care. 2003; 7(5): 1-3.
- R Ramaswamy, KH Narsinh, A Tuan, et al., Systemic air embolism following percutaneous lung biopsy, Seminars in interventional radiology, Thieme Medical Publishers. 2014; 375-377.
- MA Mirski, AV Lele, L Fitzsimmons, et al. Diagnosis and treatment of vascular air embolism. The Journal of the American Society of Anesthesiologists. 2007; 106(1): 164-177.
- W Bou-Assaly, P Pernicano, E Hoeffner. Systemic air embolism after transthoracic lung biopsy: a case report and review of literature. World Journal of Radiology. 2010; 2(5): 193-196.
- ARM Tahir, N Agussaiful, SA Hisham, et al. Drug utilisation evaluation study on patients with diabetes mellitus among Rohingya refugees in IMARET mobile clinic. Malays J Med Health Sci. 2020; 16: 51-57.
- KW Lee, NK Devaraj, SM Ching, et al. Effect of SGLT-2 inhibitors on non-alcoholic fatty liver disease among patients with type 2 diabetes mellitus: Systematic review with meta-analysis and trial sequential analysis of randomized clinical trials. Oman medical journal. 2021; 36(3): e273.
- A Norhammar, L Mellbin, F Cosentino. Diabetes: Prevalence, prognosis and management of a potent cardiovascular risk factor. European journal of preventive cardiology. 2017; 24(_suppl): 52-60.
- A Brea, J Puzo. Non-alcoholic fatty liver disease and cardiovascular risk. International journal of cardiology. 2013; 167(4): 1109-1117.
- J Dib, AJ Boyle, M Chan, et al. Coronary air embolism: a case report and review of the literature. Catheterization and cardiovascular interventions. 2006; 68(6): 879-900.
- S Romagnoli, Z Ricci, D Quattrone, et al. Accuracy of invasive arterial pressure monitoring in cardiovascular patients: an observational study. Crit. Care. 2014; 18(6): 1-11.
- R Sehra, K Underwood, P Checchia. End tidal CO2 is a quantitative measure of cardiac arrest. Pacing and clinical electrophysiology. 2003; 26(1p2): 515-517.
- MG Angelos, DJ Debehnke, JE Leasure. Arterial pH and carbon dioxide tension as indicators of tissue perfusion during cardiac arrest in a canine model. Critical care medicine. 1992; 20(9): 1302-1308.
- C Sandroni, P De Santis, S D’Arrigo. Capnography during cardiac arrest. Resuscitation, 2018; 132: 73-77.
- S Fukuda, DS Warner. Cerebral protection. BJA: British Journal of Anaesthesia. 2007; 99(1): 10-17.